New results of Land Green Technology research - in the article "Impact of nano-nutri-priming and hydro-priming on Shiroudi (Oryza sativa) traits in different pH environments"
ABSTRACT
Nanoparticles (NPs) in agriculture present new opportunities to modify seed behavior and induce physiological, metabolic, and morphological changes, particularly through innovative seed priming methods like nano-nutri-priming (Nnp). This study compared Nnp and hydro-priming (Hp) techniques on Shiroudi rice (Oryza sativa) under varied pH conditions. The Nnp method, using a nutrient mixture of boron, molybdenum, and manganese, showed significant enhancements under neutral pH, increasing germination percentage by 7.7%, vigor index-2 by 37.5%, and dry matter accumulation by 16.6% compared to the control. However, in acidic and alkaline conditions, the efficacy of NPs was inhibited, possibly due to pH-induced suppression of NP activity. In contrast, hydro-primed Shiroudi grown in acidic pH exhibited notable improvements, with germination increasing by 15.4%, vigor index-1 by 25%, and dry matter accumulation by 30.4%. Notably, root fresh weight increased with Nnp regardless of pH, and root dry weight was enhanced under neutral and alkaline conditions. Stepwise multiple regression analysis identified shoot dry weight as a strong predictor of overall dry matter accumulation, with a highly significant positive correlation (R = 0.72**), highlighting its potential as a valuable indicator for plant growth and biomass accumulation.
Keywords: rice, seed priming techniques, nano nutrient mixture, pH levels, physiological response
INTRODUCTION
Half of the world's population relies on rice as a core component of their diet, providing up to 50% of their daily caloric intake, making it essential for global food security (Fahad et al., 2019; Muthayya et al., 2014). However, climate change over the past decade has significantly affected cereal crops, particularly rice, leading to challenges that contribute to global food shortages (Mazhar et al., 2022). Rising temperatures and an intensified hydrological cycle are impacting seed germination and plant development, with the ability of seeds to germinate quickly and establish vigorous seedlings being crucial for successful crop production (Eskandari, 2013). Ranmeechai et al. (2022) emphasize that seed germination and vigor are critical to enhancing seedling growth and ultimately crop yield.
Germination begins with the absorption of water by mature, dry seeds (imbibition) and concludes with the elongation of the embryonic axis, typically the radicle, which penetrates the seed coat (Rajjou et al., 2012). During this process, α-amylase plays a key role in starch degradation, initiating the breakdown of native starch granules into smaller glucose fragments, which are essential for energy production during germination (Kato-Noguchi and Macías, 2005; Mishra and Dubey, 2008). Increasing α-amylase activity is vital for enhancing plant growth, especially in carbohydrate metabolism (Mahakham et al., 2017). Furthermore, priming, or hardening, has been shown to influence enzyme activity, aiding in the mobilization of sugars and improving seedling vigor (Farooq et al., 2006a). Hydropriming, a cost-effective priming technique, enhances germination by facilitating water absorption and boosting energy-related metabolites, which induce physiological changes in organic compounds, sugar levels, and ion accumulation, resulting in improved germination and stress resistance (Alvarado et al., 1987; Ellouzi et al., 2023).
In recent years, nanotechnology has shown promise in addressing environmental challenges brought on by climate change (Mazhar et al., 2022). Nano-priming, a novel seed priming method, enhances seed germination, growth, and yield while increasing resistance to various environmental stresses. Nanoparticles (NPs) in seed priming improve electron exchange and surface reactions in plant cells, boosting plant growth (Nile et al., 2022). NPs are being optimized for plant growth, stress resilience, and overall productivity due to their unique physico-chemical properties (Ioannou et al., 2020; Pereira et al., 2021; Singh et al., 2021; Sharma et al., 2022). Nano-priming has been shown to improve seed germination, seedling growth, stress tolerance, and crop yield by altering seed metabolism, regulating ROS homeostasis, and interacting with phytohormones (Tripathi et al., 2017; Wang et al., 2020; Ye et al., 2020; Pereira et al., 2021). Nutrient priming is another established technique, involving the application of osmotic or nutrient-rich solutions to seeds to enhance nutrient levels, improve stress resistance, and promote plant growth (Kumar et al., 2020; Liu et al., 2022; Ma et al., 2024; George et al., 2024). The inclusion of NPs in nutri-priming is particularly beneficial, as NPs' stability and adsorption properties enhance seed performance across various environmental conditions (Lee and Kasote, 2024).
Research on nano-priming has demonstrated its ability to improve water and nutrient uptake by creating nanopores and inducing aquaporin gene expression, as well as stimulating seed germination and early seedling growth through ROS production and enzyme activation (Gupta et al., 2024). Underlying that in field conditions, despite promising results, the unintended release of metal-based NPs into the environment raises concerns about their potential phytotoxic effects (Nowack and Bucheli, 2007). NPs can interact with plants, leading to uptake and accumulation that influence their transport and fate in the ecosystem (Dietz and Herth, 2011). NPs within the size range of cell wall pores can cross the wall and reach the plasma membrane, with smaller NPs entering plant cells more easily than larger ones, which cannot penetrate the cells (Navarro et al., 2008; Verano-Braga et al., 2014).
While some NPs may cause adverse effects such as inhibiting germination or inducing phytotoxicity (Pelegrino et al., 2020; Falco et al., 2020), others, including Fe, Ag, Zn, Mn, and Cu, have been reported to enhance crop growth when used as pre-sowing agents (Sharma et al., 2021). The biological responses to NPs depend on their physical and chemical properties, such as size, zeta potential, and concentration (Pérez-de-Luque, 2017; Acharya et al., 2019).
This study aims to explore an alternative to conventional hydropriming by employing a nano-mixture containing boron, molybdenum, and manganese as a nano-nutri-priming agent. The priming process, a pre-field application, uses NPs in very low doses to act as stimulators, minimizing any negative environmental impact or phytotoxicity. The objective is to evaluate the effects of this nano-nutri-priming on the physiological and phenotypic traits of Shiroudi rice (Oryza sativa) under varying pH conditions.
MATERIALS AND METHODS
Characterization of B, Mo and Mn-based NPs
Nanoparticles of boron (B NPs), molybdenum (Mo NPs), and manganese (Mn NPs) were synthesized using the flow-levitation method described by Leipunsky et al. (2019). Transmission electron microscopy (TEM) with a JSM-7401F scanning electron microscope (JEOL Ltd., Japan) operating at 1 kV was employed to determine their morphology and size. Microphotographs were analyzed using Micran 25, with measurements conducted on a minimum of 1000 particles. X-ray crystallography was performed using an ADP-1 unit (Advanced Non-Destructive Testing Technologies, Russia) with a cobalt tube as the radiation source, and the interference peaks were analyzed using Match 3.8.0.137 to ascertain the phase composition.
The Laboratory of Nano- and Microstructural Materials Science at the V.L. Talrose Institute for Energy Problems of Chemical Physics, part of the Semenov Federal Research Center for Chemical Physics, Russian Academy of Sciences, investigated the physical and chemical characteristics of the nanoparticles. The NPs exhibit monocrystalline structures with a uniform spherical shape, coated with a translucent oxide film. The average diameter of Mn NPs is 25.0 ± 0.8 nm, for B NPs it is 134 ± 12 nm, and for Mo NPs it is 51.0 ± 2.1 nm. X-ray phase analysis indicated that Mo NPs consists of 64% crystalline metal phase and 36% dimolybdenum carbide. Mn NPs contains 92-96% crystalline phase and 4.0-8.0% manganese oxide. B NPs showed an amorphous structure and comprised 92.5% boron, 7.2% carbon, and 0.3% oxygen.
Seed characteristics
Land Green and Technology provided seeds of the “Shiroudi” cultivar of rice. Shiroudi is a transgenic variety resulting from a cross between two rice cultivars: Khazar, known for its high yield and resistance to blast disease, and Daylamani Tarom, which is renowned for its excellent cooking quality. This variety underwent testing by the Seed and Plant Improvement Institute of Iran and exhibits resistance to rice blast, tolerance to stem borer, and moderate susceptibility to sheath blight disease. It possesses several notable characteristics, including a moderate amylose content (23.5%), suitable plant height (106 cm), long panicle length (27 cm), high weight of 1000 grains (26 g), early maturity (124 days), and high yield (6.5-7 t/ha). Shiroudi was officially registered as a new rice cultivar in Iran in 2007 under this name (Mohadesi et al., 2009).
Preparation of nano-nutri-priming solution
The nano-nutri-priming mixture of B NPs, Mo NPs, and Mn NPs was prepared as follows: A polymer blend was created by mixing 1% w/w Na2-carboxymethyl cellulose (Akzo Nobel, Netherlands), 2.5% polyethylene glycol-400 (NikaChem, RF), and 0.037% Na2-EDTA (China, Lot No. 20160379). Rhodamine 6G (0.002%, “Lenreaktiv”, RF) was included for visualization, and Benomyl (TARI, Taiwan) for fungicidal purposes. The concentration of nanoparticles (NPs) used is intentionally low, as their role is to act as a stimulant in seed priming. The dose of NPs was selected based on a previous study by Davydova et al. (2019). They were dispersed in water using a Scientz JY 92-IIN ultrasonic disintegrator, set to operate at 44 kHz for 30 s with 30-s intervals, repeated three times under ice cooling. This process aimed to achieve concentrations of [B]: 10-4 mg/L, [Mo]: 10-6 mg/L, and [Mn]: 10-6 mg/L, which were then added to the polymer blend during sonication.
Seed priming and germination
The entire experiment was conducted under laboratory conditions using rice seeds of the “Shiroudi” variety. Each batch, consisting of 50 seeds designated for nanoparticle (Nnp) treatment, was immersed in a solution containing 10 ml of NP suspension, 0.05 ml of EDTA, and 0.1 ml of benomyl. Batches designated for hydro-priming (Hp) were soaked in distilled water. After immersion, all seeds were rinsed three times with distilled water and then dried back to their original weight.
The priming process was conducted under controlled experimental conditions in a thermostat set to 40°C, in complete darkness, at 75% humidity, with continuous fan operation for 24 h. After priming, seeds were divided into treatment groups (each containing 50 seeds) based on the medium's pH level:
- T0 (Hp-pH5.5)
- T1 (Nnp-pH5.5)
- T2 (Hp-pH7)—used as the experimental control
- T3 (Nnp-pH7)
- T4 (Hp-pH8)
- T5 (Nnp-pH8)
Throughout the whole experiment, the treated seeds were placed in a constant temperature incubator at 32°C ± 1°C and 75% relative humidity. During the first four days, seeds were kept in total darkness, followed by lighting conditions from day five to day seven. Germination was assessed using the roll method (GOST 12038-84). The experiment was repeated twice for reliability.
Physiological parameters
To proceed with statistical analysis, physiological parameters were calculated using the following equations: Imbibition, the initial stage of germination, is characterized by a rapid increase in seed water uptake. To assess this, seed weight was recorded before priming (dry weight) and again after 24 h (imbibed weight). Imbibition percentage was then calculated using Equation 1, following the method of Baskin et al. (1998).
dry seed weight
(Eq.1)
The germination percentage, representing the proportion of seeds that successfully sprout within a specific time frame, was calculated on day three using Equation 2, in accordance with the ISTA 2006 standard.
total seeds
(Eq.2)
Seed vigor is a key quality parameter that provides insight into the potential field performance of a seed lot. Accordingly, two vigor indices were calculated based on the method of Abdul-Baki and Anderson (1973), using Equations 3 and 4.
(Eq.3)
(Eq.4)
Total dry matter represents the cumulative result of all plant processes over space and time. The rate of dry matter accumulation depends on total incident solar radiation, the crop canopy's absorption of this radiation, and the efficiency of converting absorbed radiation into plant dry matter. Due to its significance, dry matter accumulation was calculated using Equation 5, as described by Buckmaster (2005).
Fresh weight of seedling
(Eq.5)
Starting from the third day after sowing (DAS) until the end of the experiment on the 7th day, phenotypic parameters such as shoot length (SL), germinal root length (RL), number of adventitious roots (nAR), and average length of adventitious roots (LAR) were measured daily. On the 7th day (DAS), destructive sampling was performed to measure the fresh weight of shoots (FWS) and roots (FWR). Subsequently, the seedling fresh weight (SFW) was calculated, along with the measurement of the dry weight of shoots (DWS) and roots (DWR), and determination of the seedling dry weight (SDW). Each treatment had 15 replicates. Length and fresh biomass of shoots and roots were measured immediately using a 1/10,000 scale and meter ruler after harvest. Dry weights were measured after drying in an oven (1 h at 105°C and 48 h at 65°C).
Statistical analysis
Data analysis was performed using IBM SPSS Statistics 20 software. A one-way ANOVA followed by Duncan's Multiple Range Test (DMRT) was employed to analyze the physiological parameters, including the fresh and dry weights of the individual parts as well as the entire seedlings, while Repeated Measures ANOVA was applied to analyze the dynamic progression of phenotypic characteristics. Seed imbibition was analyzed using a t-test. Results were presented as mean ± standard error (SE), with a significance level set at p ≤ 0.05 for the DMRT. Pearson's correlation was utilized to examine relationships between physiological and phenotypic characteristics, while stepwise multiple regression was conducted to determine associations between dry matter accumulation and the fresh and dry weights of shoots and roots. All graphs were created using Microsoft Excel 2010.
RESULTS
Effect of treatments on physiological characteristics
An independent T-test showed no significant difference in imbibition% between the Hp and Nnp methods. However, Figure 1a and b illustrates distinct variations in germination% and vigor indexes across different media pH levels. The highest germination%, reaching 100%, was achieved in acidic pH conditions, regardless of seed priming method, with a similar result in alkaline pH when using Hp. In neutral pH, Nnp improved germination by 6.66% compared to Hp. Therefore, germination% is significantly influenced by media pH, with Nnp yielding significantly better results than Hp only in neutral media. For Vigor index 1, calculated using Equation 3, values were significantly higher in acidic media, with no notable difference between the priming methods, reaching 14.77 for Hp and 15.25 for Nnp. Similarly, in alkaline media, Hp outperformed the control (12.13) with a Vigor index of 14.15. For Vigor index 2, calculated using Equation 4, the lowest values in acidic media were observed with Nnp (0.0062) and Hp (0.0075). Conversely, the highest Vigor index 2 was recorded in neutral media with Nnp (0.66). At alkaline pH, Nnp's Vigor index 2 was lower by 0.02 compared to Hp.
Values are means ± SD, for each indicator, means followed by the same letter are not significantly different at p < 0.05 according to Duncan's multiple range test (n = 15)
Effect of treatments on seedlings weight and dry matter accumulation
An interesting trend was observed in root fresh weight, with a significant decrease as media pH increased. Within the same pH media, Nnp significantly improved root fresh weight compared to Hp, with the highest root weight recorded in acidic media when rice seeds were treated with Nnp (T1 Nnp-pH5.5: 0.031 g). For the shoots, Nnp significantly increased fresh weight in alkaline media compared to Hp, while the opposite was observed in acidic conditions. In neutral conditions, no significant differences were noted between priming methods. The heaviest shoots among treatments were: T0 Hp-pH5.5 (0.03 g), T2 Hp-pH7 (0.029 g), and T3 Nnp-pH7 (0.028 g) (Fig. 2a). The dry weight of roots was significantly affected by Nnp in neutral and alkaline media, where it notably exceeded that of Hp in the same conditions (T2 Hp-pH7: 0.0025 g vs. T3 Nnp-pH7: 0.0031 g; T4 Hp-pH8: 0.0021 g vs. T5 Nnp-pH8: 0.0023 g). The highest root dry weight was recorded in acidic media with Hp (T0 Hp-pH5.5: 0.0036 g). Shoot dry weight remained consistent at 0.004 g across treatments, except in T1 Nnp-pH5.5 and T2 Hp-pH7, where it significantly decreased to 0.003 g (Fig. 2b).
As illustrated in Figure 3, broad screening reveals that the fresh weight of seedlings exhibited the same pattern as the fresh weight of roots, wherein Nnp significantly improved this parameter compared to Hp in the same media's pH, indicating that it has a stronger impact on the total seedling weight. Regarding the seedlings dry weight, all treatments showed a significantly higher dry weight of seedlings, except for T4 Hp-pH8, where it was comparable to the control. It is noteworthy that in acidic pH, Nnp showed a significant adverse effect on this parameter. However, in neutral and alkaline media, Nnp either significantly improves seedlings dry weight or slightly enhances it without significance, respectively. The highest dry matter accumulation is observed in acidic and alkaline media with Hp, while in the same media with Nnp, it decreases significantly by 4.57% and 2.27%, respectively.
Values are means ± SD, for each indicator, means followed by the same letter are not significantly different at p < 0.05 according to Duncan's multiple range test (n = 15)
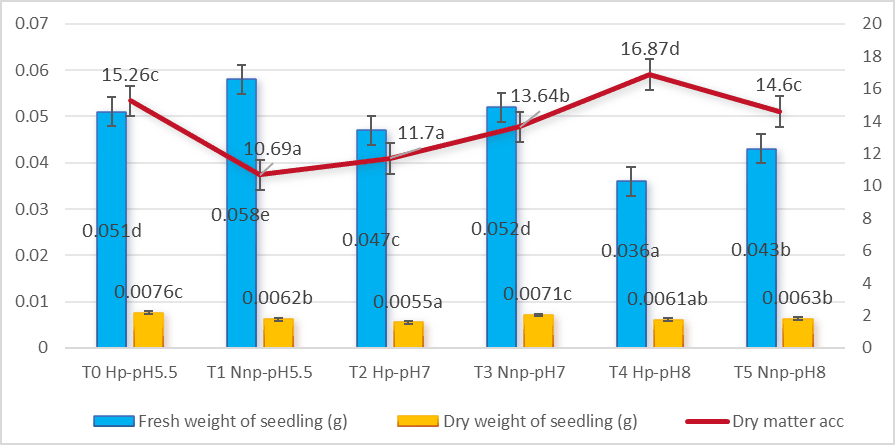
Values are means ± SD, for each indicator, means followed by the same letter are not significantly different at p < 0.05 according to Duncan's multiple range test (n = 15)
Effect of treatments on seedlings dynamic phenotypic characteristics
Table 1 presents the results from the Repeated Measures ANOVA, which indicate that the combined factor “Hydro-priming-media pH (Hp-pH)” significantly affected shoot length and the average length of adventitious roots, while root length and the number of adventitious roots were not significantly impacted. The interaction of “t*Hp-pH” showed significant effects on shoot length, the number of adventitious roots, and the average length of adventitious roots, but had no impact on root length.
Regarding the factors “Nnp-pH” and “t*Nnp-pH,” neither the separate nor the combined effects significantly impacted shoot length, root length, or the number of adventitious roots. The only parameter affected in both cases was the average length of adventitious roots.
Effect | SL | RL | nAR | aLAR |
---|---|---|---|---|
Treatment (Hp-m) | 0.003 | 0.134 | 0.159 | <0.001 |
Treatment (Nnp-m) | 0.79 | 0.064 | 0.191 | <0.001 |
t*Hp-m | <0.001 | 0.15 | <0.001 | <0.001 |
t*Nnp-m | 0.281 | 0.48 | 0.180 | 0.002 |
Repeated Measures ANOVA was employed to examine the impact of the independent variable Hp-m; Nnp-m; t*Hp-m and t*Nnp-m on the repeated measures of the dependent variable
SL: Shoot length,
RL: Root length,
nAR: number of adventitious roots,
aLAR: average length of adventitious root.
p < 0.05
Figure 4 illustrates the dynamic growth patterns of seedlings under various treatments. A representative seedling from each treatment was selected to highlight the variations specific to each condition. Overall, Nnp exhibits superior performance compared to Hp, though the differences are not consistently statistically significant. Notably, Nnp appears to facilitate faster seedling development.
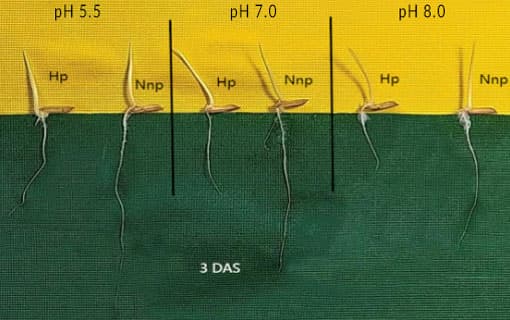
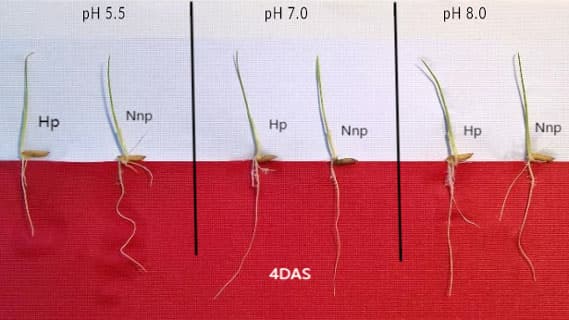
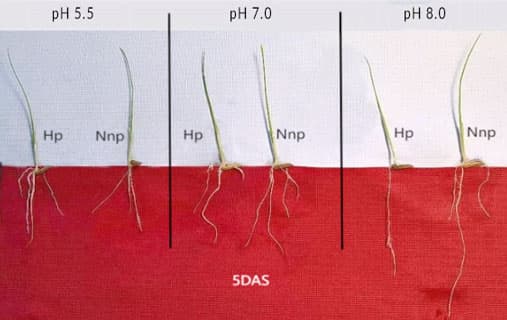
Correlation and stepwise multiple regression analysis
Vigor index-2 is directly related to seedlings dry weight, which in its turn is strongly and highly significantly correlated (0.848**) with the dry weight of shoots and moderately, yet highly significantly, correlated (0.545**) with the dry weight of roots. Consequently, Vigor index-2 exhibit a primarily strong and highly significant correlation (0.792**) with the dry weight of shoots, rather than the moderate positive and highly significant correlation (0.573**) with the dry weight of roots. Seedling fresh weight showed a highly significant strong correlation (0.796**) with the fresh weight of shoots, but an even stronger highly significant correlation (0.873**) with the fresh weight of roots. Dry matter accumulation demonstrated a strong positive and highly significant correlation (0.720**) with the dry weight of shoots and a weak negative highly significant correlation (-0.300**) with the dry weight of roots (Table 2).
Based on the findings from stepwise multiple regression (Table 3), the most explanatory models, specifically models 3 (R2: 0.90) and 4 (R2: 0.97) indicate that dry matter accumulation is predominantly influenced by the dry weight of shoots. In particular, an increase in the dry weight of shoots correlates with an enhancement in dry matter accumulation, as evidenced by the positive coefficient. These outcomes suggest the potential utility of utilizing both fresh and dry weights of rice seedlings parts to forecast dry matter accumulation, thereby facilitating insights into plant growth and metabolic efficiency.
VI-1 | |||||||||
VI-2 | R | .224* | |||||||
P | 0.034 | VI-2 | |||||||
FWS | R | -0.17 | .411** | ||||||
P | 0.109 | <.001 | FWS | ||||||
FWR | R | 0.133 | .280** | .399** | |||||
P | 0.211 | 0.008 | <.001 | FWR | |||||
SFW | R | -0.003 | .404** | .796** | .873** | ||||
P | 0.98 | <.001 | <.001 | <.001 | SFW | ||||
DWS | R | -0.017 | .792** | .225* | -0.166 | 0.01 | |||
P | 0.875 | <.001 | 0.033 | 0.117 | 0.927 | DWS | |||
DWR | R | .221* | .573** | .583** | .730** | .792** | 0.017 | ||
P | 0.036 | <.001 | <.001 | <.001 | <.001 | 0.874 | DWR | ||
SDW | R | 0.103 | .968** | .498** | .248* | .429** | .848** | .545** | |
P | 0.333 | <.001 | <.001 | 0.018 | <.001 | <.001 | <.001 | SDW | |
DMA | R | 0.137 | .466** | -.376** | -.611** | -.603** | .720** | -.300** | .445** |
P | 0.197 | <.001 | <.001 | <.001 | <.001 | <.001 | 0.004 | <.001 |
*Correlation is significant at the 0.05 level (95%) (2-tailed).
**Correlation is significant at the 0.01 level (99%) (2-tailed).
SL: seedling length, VI-1: vigor index-1, VI-2: vigor index-2, FWS: fresh weight of shoots, FWR: fresh weight of roots, SWR: seedling fresh weight, DWS: dry weight of shoots, DWR: dry weight of roots, SDW: seedling dry weight, DMA: dry matter accumulation
Dependent | Predictors | Model equation | Adj. R2 | Model n |
---|---|---|---|---|
DMA | DWS | DMA = 2186 × DWS + 5.8 | 0.51 | Model 1 |
DMA | DWS, FWS | DMA = 2572 × DWS - 329 × FWS + 13.2 | 0.82 | Model 2 |
DMA | DWS, FWS, FWR | DMA = 2313 × DWS - 244 × FWS - 148 × FWR + 15 | 0.90 | Model 3 |
DMA | DWS, FWS, FWR, DWR | DWA = 2254 × DWS - 330 × FWS - 275 × FWR + 2144 × DWR + 14.2 | 0.97 | Model 4 |
DMA: dry matter accumulation, DWS: dry weight of shoots, FWS: fresh weight of shoots, FWR: fresh weight roots, DWR: dry weight of roots
DISCUSSION
Environmental conditions are critical to rice establishment, with pH playing a significant role in plant growth and chemical composition. Earlier studies have highlighted that maintaining a pH range of 5.5 to 6.5 is optimal for healthy growth and efficient nutrient uptake in rice plants. Conversely, extreme pH levels significantly inhibit growth: at pH 3.5, root systems are severely stunted with inhibited lateral growth, while at pH 8.5, both root development and leaf health deteriorate, as evidenced by chlorosis (Alam, 1981). Building on these findings, this study evaluates the effectiveness of Hp and NnP in mitigating the adverse effects of abiotic stress under controlled laboratory conditions.
Seed imbibition, a critical process that reactivates metabolism and hydrolytic enzymes, facilitates germination (Pompelli et al., 2023). While our findings showed no significant differences in the imbibition process between Hp and NnP, subsequent developmental stages revealed substantial interactions between the priming method and pH levels, with notable variations in performance.
It is well established that pH influences most chemical and biological processes (Enayati Ahangar et al., 2022). Hotze et al. (2010) demonstrated that pH significantly affects the physical and chemical properties of nanoparticles (NPs), including surface charge, availability, and oxidative dissolution. While specific studies using boron (B), molybdenum (Mo), and manganese (Mn) nanoparticles under varying pH conditions are limited, analogous research on silver nanoparticles (AgNPs) suggests that similar pH-induced modifications likely occur (Fernando and Zhou, 2019). Moreover, acidic conditions inhibit enzyme synthesis and activity, dissolve seed coats, or promote pathogen growth (Vleeshouwers et al., 1995). On the other hand, moderately acidic conditions enhance enzymatic activity, facilitating germination. In our case, germination in acidic media reached 100%, regardless of priming method. However, germination at neutral pH improved significantly with NnP compared to Hp, consistent with studies showing that NnP improve germination by enhancing enzymatic activity and improves seed quality (Barik et al., 2022).
Though we did not directly measure enzymatic activity, previous studies suggest that the elements in our NnP mixture likely play a role in enhancing metabolic processes. B-based nutri-priming has been shown to activate key enzymes like α-amylase and starch phosphorylase, enhancing sugar availability for protein synthesis and accelerating germination (Pawar and Laware, 2018; Rasool et al., 2019). Similarly, Mn promotes photosynthesis, ATP production, and RuBP enzyme regeneration (Salehi et al., 2023), while also boosting DNA polymerase activity, crucial for DNA repair in meristematic tissues (Kirby et al., 2012; Alejandro et al., 2020). B and Mn in non-nano forms have demonstrated significant benefits for seed germination and early development (Tamindžić et al., 2024).
The role of NP size and concentration in influencing seedling traits is well-documented. Smaller NPs at lower concentrations are more effective in root tissues, as reported by Lin and Xing (2008) and Geisler-Lee et al. (2013). Thuesombat et al. (2014) showed that low-concentration AgNPs remain localized in root tissues, while larger NPs at higher doses can translocate to shoots. Our findings suggest that NnP likely remained localized in root zones, which explains its significant impact on root parameters but limited influence on shoot growth.
Rice typically thrives in soils with a pH of 5.5-6.5, though different varieties exhibit varying tolerances. High concentrations of H+ ions suppress root growth, reducing the development of fine roots and nutrient uptake (Zhang et al., 2015). Acidic conditions also inhibit metabolic processes, photolysis, and water uptake (Kamaluddin and Zwiazek, 2004). Hp has been shown to mitigate some of these stresses, promoting sugar mobilization and enzymatic activity (Farooq et al., 2006b). Our results demonstrate that Hp alleviates the adverse effects of pH to some extent, but NnP offers greater improvements in root parameters and dry weight under neutral and alkaline conditions.
The phenotypic responses of rice seedlings to NPs vary across studies. While some report significant enhancements in root and shoot growth (Mahakham et al., 2017; Cyriac et al., 2020), others note inhibitory effects at higher NP concentrations (Prasad et al., 2012). In our study, NnP effectively mitigated the negative effects of pH on root length and dry weight, highlighting its potential as a stress alleviation method.
Seedling vigor, a key agronomic trait, encompasses metrics such as germination rate, dry matter accumulation, and metabolic efficiency, which are predictive of field performance and yield (Wang et al., 2010; Ronanki et al., 2017). Primed seeds exhibit enhanced antioxidant systems and metabolic activity, contributing to improved stress tolerance and resilience during early establishment (Chen and Arora, 2013). Our findings reinforce the importance of priming methods in optimizing seedling performance under abiotic stress, particularly in challenging pH environments.
By promoting robust seedling development and stress resilience, Nnp emerges as a promising technique for enhancing rice establishment and productivity in soils with suboptimal pH.
CONCLUSIONS
This paper presents a comparative study between NnP and Hp of rice seeds grown under varying pH conditions in a controlled laboratory setting. Both methods were evaluated as pre-field transplantation techniques aimed at improving seedling establishment and performance in the field. Notably, the NnP approach is eco-friendly, as it involves the use of nanoparticles (NPs) in very low doses, minimizing their environmental impact by avoiding direct application into the ecosystem.
The results demonstrate that NnP is a promising strategy for enhancing rice production in soils with unfavorable pH, particularly neutral pH conditions. It was found to significantly improve the physiological and phenotypic traits of rice seedlings. While extreme pH conditions suppressed some of the effects of nanoparticles, NnP still led to notable improvements in root development and shoot dry weight—key predictors of dry matter accumulation and eventual crop yield. These findings suggest that the NnP mixture, comprising boron, molybdenum, and manganese NPs, may specifically target the root system and shoot metabolism, enhancing growth through mechanisms influenced by NP size and concentration. The study highlights the potential of NnP to improve rice tolerance to pH stress by stimulating key metabolic pathways. However, it also emphasizes the need for further research to elucidate the cellular and enzymatic modifications driving these enhancements. Future studies should focus on exploring the behavior of primed seedlings under open-field conditions, while carefully monitoring the fate and pathways of nanoparticles in the environment. This work underscores the potential of NnP as a sustainable and effective approach to address challenges in rice cultivation under adverse soil conditions.
© Land Green and Technology Co., Ltd., Taipei, Taiwan